With global food demand expected to surge by up to 56% from 2010 to 2050, and crop yields under threat from climate change at an estimated 11% loss per decade, agriculture faces mounting challenges. Addressing these pressures, the United Nations’ Sustainable Development Goal 2.4 calls for sustainable and resilient farming practices that can adapt to climate change and natural disasters while maintaining ecosystem health and soil quality. Technologies such as smart irrigation and advanced plant health monitoring are emerging as essential tools in supporting this shift toward sustainable food production.
One promising solution is Terahertz (THz) imaging. This technology has recently gained attention as a non-invasive, in vivo technique for assessing plant health, particularly for detecting early water stress and signs of parasitic activity on leaves. THz imaging operates between 0.1 and 10 THz, where the frequency range interacts with hydrogen bonds in water molecules and other leaf constituents, enabling real-time imaging of plant status.
In this case study, we explore the potential of using Rydberg atoms for THz imaging on autonomous robotic platforms. By integrating these advanced sensing technologies, we aim to provide insights on how autonomous systems can monitor crop health with greater precision, supporting more resilient and adaptive agricultural systems.
Existing Technical Solutions
The graphic below provides a comprehensive overview of THz sensing technologies, including various imaging techniques, modalities, sources, and detectors, alongside the specific configuration selected for this study.
One prominent approach is active THz imaging using a multiplier-based source, which emits THz radiation toward the target and detects the reflected signals through a Rydberg atom-based system. This system converts THz radiation into optical signals via atomic vapor, which are then captured by a standard camera to produce an image. Multiplier-based sources are especially suited for mobile platforms due to their compact design and efficient generation of THz waves by frequency multiplication.
The visual highlights the positioning of the Rydberg Detector relative to other detectors typically used in THz imaging systems.
Although current Rydberg Detector prototypes do not yet match the sensitivity, size, weight, and power (SWaP) efficiency of commercial detectors, particularly bolometers, they offer unique advantages. With development, Rydberg Detectors hold promise for enhanced sensitivity and reduced SWaP, allowing for competitive integration on mobile platforms. Their primary advantage lies in higher frame rates, as they avoid the thermal lag that limits bolometers, enabling faster and more responsive imaging capabilities.
Quantum Sensing State-of-the-Art
Recent breakthroughs in THz imaging have been propelled by pioneering research on Rydberg atom-based systems, notably from work conducted at Durham University.
One notable development is detailed in the paper, „Full-Field Terahertz Imaging at Kilohertz Frame Rates Using Atomic Vapor.“ This study presents a high-speed THz imaging system that leverages caesium atoms in a vapor cell. When excited to Rydberg states and exposed to a THz field, these atoms transition in ways that emit optical photons, which are then captured by a camera. The result is full-field imaging with remarkable frame rates of up to 3000 frames per second. This represents a significant advancement over conventional THz imaging technologies, providing near diffraction-limited spatial resolution and a minimum detectable power of 190 fW per pixel.
Further optimization efforts are described in the thesis, „Optimizing a Rydberg Atom-based Terahertz Imaging System“ by Matt Jamieson. This work achieved a 38% improvement in fluorescence signal strength and enhanced the system’s spatial resolution, increasing its sensitivity to variations in THz fields. These improvements make the system more effective and practical for real-world applications.
In collaboration with industry, a compact laser system was also developed to support this type of Rydberg atom-based instrument, underscoring the potential for practical deployment in advanced THz imaging solutions.
Application Needs
For effective plant health monitoring, particularly in the context of moisture detection, specific characteristics of both plant physiology and THz imaging are critical for assessing and comparing imaging technologies.
THz frequency range for leaf moisture imaging (THz) | 0.1 – 3.5 |
Typical leaf thickness (mm) | 0.1 – 0.8 |
Amount of water in leaf (%) | 58 – 85 |
Absorption coefficient of water (cm-1) | 120 – 550 |
Refractive index of water | 4 – 2.1 |
Achievable feature resolution (µm) | 1000 – 100 |
Concept of Operation
In THz reflection imaging for plant health analysis, a robotic arm-mounted THz imaging system precisely positions itself close to a target leaf to capture high-quality images. The system emits a THz beam, akin to a camera flash, which reflects off the surface of the leaf. To optimize signal clarity, the leaf is positioned orthogonally to the imaging system, allowing the maximum amount of reflected THz power to reach the detector. This close proximity reduces optical depth and minimizes interference from reflections of other leaves behind the target.
A commercially available THz camera serves as the core imaging component. The system integrates a lens with a THz source, additional optics, a beam splitter, and an objective lens to focus the beam precisely on the leaf’s surface. Using a multiplier source providing 1 mW of power and a leaf reflectivity of approximately 10%, the system collects about 100 µW of reflected power. This power is then distributed across a 384×288 pixel array, enabling detailed imaging of plant moisture and structural features critical for assessing plant health.
Recommendation
THz imaging for in-vivo plant leaf analysis is still emerging, and while it holds promise, there is uncertainty about its competitiveness with established methods like optical and hyperspectral imaging. These traditional techniques are well-developed, and it is yet to be determined if THz imaging will offer significant advantages in terms of practical insights and usability.
Our findings also suggest limited synergy between THz imaging and robotic platforms. Achieving consistent, high-quality results with THz imaging requires close and precise positioning control, which may only be feasible with complex robotic arms capable of fine adjustments.
Given these factors, we recommend staying engaged with the THz imaging and Rydberg THz imaging research communities to monitor progress as the technology matures. A key metric to track in future advancements will be the Size, Weight, and Power (SWaP) characteristics of the imaging system at a fixed spatial resolution. This will be crucial in assessing the practicality of THz imaging for field applications and its long-term viability alongside other established imaging methods.
PilQrim Consulting Team
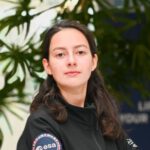
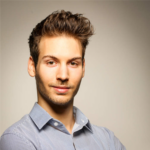
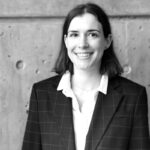