Soil degradation is projected to impact 90% of the world’s soils by 2050, while global food demand is expected to increase by up to 56% from 2010 levels. Beyond supporting crop yields, soils are vital for filtering and storing water, maintaining biodiversity, and sequestering carbon. To address these critical issues, the United Nations’ Sustainable Development Goals (SDG) 2.4 and 15.3 call for sustainable and resilient soil management practices to combat desertification, protect ecosystems, and maintain land productivity.
A key approach to promoting soil sustainability is soil component analysis, which monitors essential indicators of soil health, including chemical contamination, pesticide residues, erosion, and the capacities of soils to retain water and store carbon. Organic and inorganic soil components play distinct roles in these functions, with organic matter—though comprising less than 10% of soil composition—serving as a fundamental driver of soil properties such as fertility, water retention, nutrient availability, and carbon storage. This organic matter includes both living microorganisms and plant material, as well as decomposed elements like humic and fulvic acids.
Spectroscopy offers a powerful method for analyzing soil composition and health. This case study explores how quantum spectroscopy solutions could advance soil monitoring by providing precise, real-time data on soil constituents. Through assessing the feasibility of implementing these solutions on autonomous robotic platforms, Aardvark Sensing aims to enhance soil health monitoring and contribute to sustainable agricultural practices.
Existing Technical Solutions
The graphic below presents an overview of various infrared (IR) spectroscopy techniques, including modalities, sources, and detectors.
Among these, Fourier Transform Infrared (FTIR) spectroscopy stands out as the most widely used method for analyzing soil constituents. This technique operates by passing IR light through a sample, where specific molecular bonds absorb distinct wavelengths, generating an absorption spectrum that reveals the sample’s chemical composition.
In FTIR, a beam splitter directs IR light through the sample and towards a moving reference mirror. By overlapping this with light from a fixed path, the system captures constructive and destructive interference on the detector, creating an interferogram. This data is then processed via Fourier transform to convert time-domain information into a frequency-domain spectrum. Depending on the wavelength, high sensitivity in FTIR spectroscopy requires cryogenically cooled IR detectors.
An alternative approach uses entangled photon sources, where one photon is in the IR range and the other in the visible range. By detecting the visible photon with a standard silicon photodiode, the system can infer the corresponding IR spectral data. This technique, which eliminates the need for cryogenic cooling, could be especially beneficial for mobile platforms, offering both high sensitivity and significant reductions in size, weight, and power (SWaP).
Quantum Sensing State-of-the-art
Recent advancements in quantum infrared (IR) spectroscopy have introduced transformative techniques for high-sensitivity soil analysis.
The study, „Ultra-broadband Quantum Infrared Spectroscopy,“ showcases a system that utilizes entangled photons to achieve ultra-broadband IR measurements, covering a range from 2000 cm⁻¹ to 5000 cm⁻¹ with remarkable sensitivity. This broad spectral range enables detailed analysis of complex soil compositions, essential for monitoring organic and inorganic constituents.
Another notable advancement, „Open-Path Detection of Organic Vapours via Quantum Infrared Spectroscopy,“ applies Quantum Fourier Transform Infrared (QFTIR) spectroscopy for detecting volatile organic compounds in ambient environments. By extending the arm length, this system achieves enhanced sensitivity, successfully distinguishing between mixtures of vapors like acetone, methanol, and ethanol. This capability underscores the potential of quantum IR sensing for in-field soil monitoring applications, where detecting trace organic compounds is crucial for assessing soil health.
In addition, the research „Mid-IR Spectroscopy with NIR Grating Spectrometers“ demonstrates how commercially available near-infrared (NIR) grating spectrometers can achieve strong signal-to-noise ratios with rapid integration times, making them suitable for efficient, high-resolution soil analysis.
Finally, the paper „High-Sensitivity Quantum Sensing with Pump-Enhanced Spontaneous Parametric Down-Conversion“ highlights the use of an optical cavity to boost signal emission rates, leading to enhanced signal-to-noise ratios. This approach allows for high-resolution, mid-IR spectral analysis with a sensitivity comparable to traditional FTIR spectrometers, positioning it as a promising solution for detailed soil characterization on autonomous platforms.
These advancements underscore the rapid progress in quantum IR spectroscopy, which holds significant promise for in-field soil analysis applications, particularly in terms of high sensitivity and the potential for portable, autonomous deployment.
Application Needs
The following characteristics of soil organic composition and spectroscopy provide essential context for evaluating and comparing different imaging and sensing technologies.
Apparent absorbance of soil particles | 0.1 – 1.6 |
Typical organic component particle size (mm) | 0.1 – 0.8 |
Relevant wavenumber range (cm-1) | 1100 – 3400 |
Conclusion and Recommendation
Quantum infrared (IR) spectroscopy in the 2000–5000 cm⁻¹ range offers promising capabilities for detecting key organic bonds, such as C-H, that are essential for analyzing soil organic composition. This technology, with its low Size, Weight, and Power (SWaP) requirements and simplified detector setup, has potential for integration into autonomous robotic platforms, positioning it as a valuable tool for in-field soil health monitoring. However, some critical challenges remain before moving forward with targeted development efforts.
Key considerations include:
- Sample Handling Complexity: Quantum IR spectroscopy relies on transmission analysis, meaning that the robotic platform must be equipped to collect, prepare, and insert soil samples into the instrument. This requirement adds complexity to the system’s operation.
- Wavenumber Range Limitations: While the current range (2000–5000 cm⁻¹) is effective for certain organic compounds, many other important organic compositions absorb in the 1000–2000 cm⁻¹ range. Demonstrating capability within this lower range will be essential for comprehensive soil analysis.
- Trade-offs in System Complexity: Although quantum IR spectroscopy offers a simplified detector design, the integration of an entangled photon source may introduce additional complexity that must be balanced against these gains.
We recommend to:
- Draw Inspiration from Robotic Sample Return Missions: Investigate robotic handling concepts used in space sample return missions, as these may offer useful insights into designing effective sample collection and preparation mechanisms.
- Monitor Advances in SWaP and Performance: Keep a close watch on developments in the field, especially concerning SWaP optimization and performance improvements that could enable quantum IR systems to compete effectively with classical instruments.
- Evaluate Expansion into Lower Wavenumber Ranges: While the 2000–5000 cm⁻¹ range provides valuable insights, expanding into the 1000–2000 cm⁻¹ range will further enhance the system’s capabilities for detecting a broader array of organic compounds in soils.
PilQrim Consulting Team
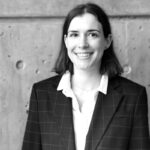
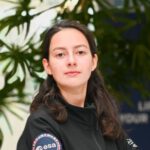
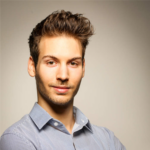